Sickle Cell Anemia — CRISPR and The Probable Hope
I have never spoken about this on any media platform, and very few in my inner circle are aware of the disease which took my mother’s life. I probably didn’t take it too seriously either; till a few years before her demise. I would see her physically struggle in the extremely cold climate during our family vacation or the daily commute to work, but the need for mental peace to ensure a good life for her family and, provide love to us all was the no. 1 priority in her books.

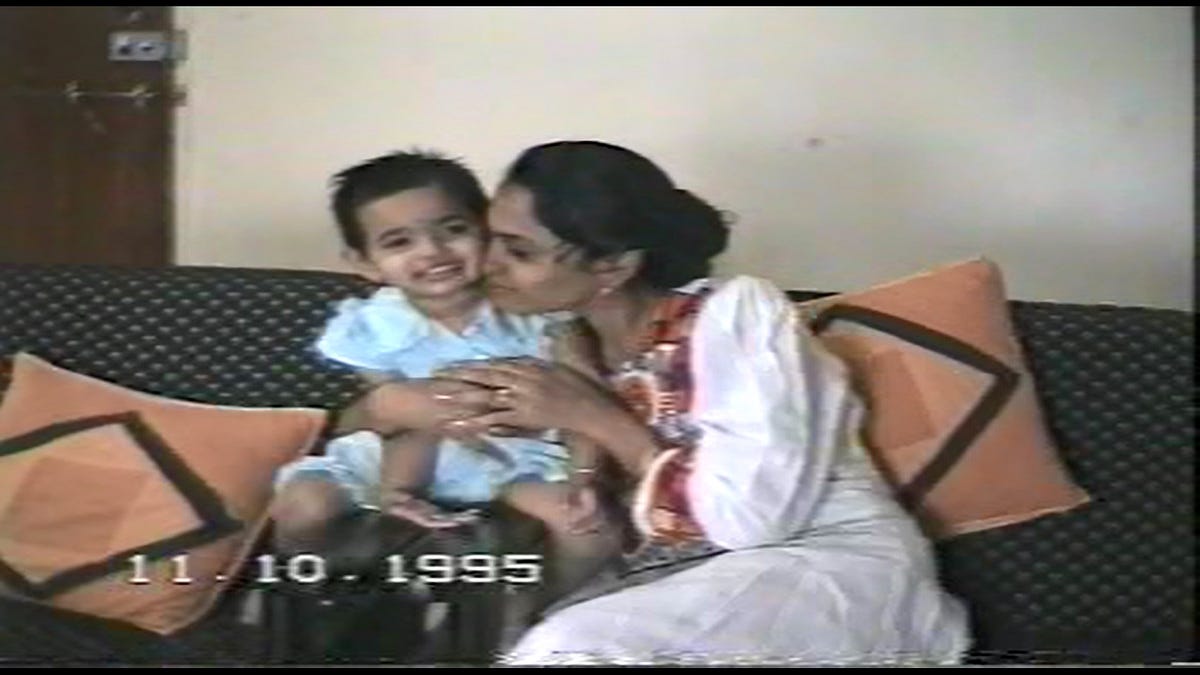
My mother suffered from Sickle Cell Anemia for the entirety of her life. A mutation in a single DNA letter is a cause for this painful and debilitating disease (Ledford, 2016). Sickle cell anemia is the most severe type of sickle cell disease. It is a group of inherited conditions that affect hemoglobin; the protein in red blood cells that transports oxygen throughout the body. A single mutation in the gene that helps to make hemoglobin alters red blood cells to a sickle, crescent-like shape. These cells die prematurely, leading to anemia, and may also get stuck in small blood vessels, blocking blood flow (Lopes, 2018).
The life expectancy with sickle cell disease had improved considerably since 1960, although Sir John Dacie described it as “essentially a disease of childhood.” He was of the opinion that the mortality rate is high and relatively few patients reach an adult life (J.V., 1960). Today, the median age at death among patients with sickle cell anemia was 42 years (for males) and 48 years (for females) (Platt, et al., 1994). My mother was 47 years young, beautiful and dynamic.
As per his 1973 review based on autopsies, Diggs (1973) estimated a median survival of 14.3 years; 20 percent of the deaths occurred in the first 2 years of life, one third happened before the fifth year of life, half between 5 and 30 years of age, and about one-sixth after the age of 30. In contrast, in the Cooperative Study of Sickle Cell Disease (CSSCD), approximately 85 percent of children and adolescents with sickle cell anemia and 95 percent of patients with sickle cell-hemoglobin C disease survived to 20 years of age (Leikin, et al., 1989). Adults, with low levels of fetal hemoglobin in their childhood, have a high probability of dying earlier than those who had high levels. The fetal hemoglobin level can, therefore, be considered an essential factor in choosing children who can opt for high-risk interventions such as bone marrow transplantation and long-term treatments. The treatments can stimulate the production of fetal hemoglobin (Platt, et al., 1994).
Now, the question that may arise is what one can do when they don’t have the resources available to carry out such extensive and expensive treatments? How can we be guaranteed that a short visit to the hospital, for an entirely different illness, may not lead to the final stage of the sickle cell disease? What if this disease, and numerous such, can be cured by tweaking the mutation; thus, offering a fruitful life, free of the illness?
CRISPR Therapeutics, founded by Rodger Novak, Emmanuelle Charpentier, and Shaun Foy in November 2013, focuses on the advancement of transformative medicines using its proprietary CRISPR/Cas9 gene-editing platform. CRISPR/Cas9 is a progressive technology that allows for precise, directed changes to genomic DNA (CRISPR Therapeutics, 2017). Over the past few years, the technology has captured the public’s imagination for its ability to edit genetic code in a way that may correct defects inside individual cells — potentially healing mutations and preventing the advent of many illnesses (Shieber, 2018).

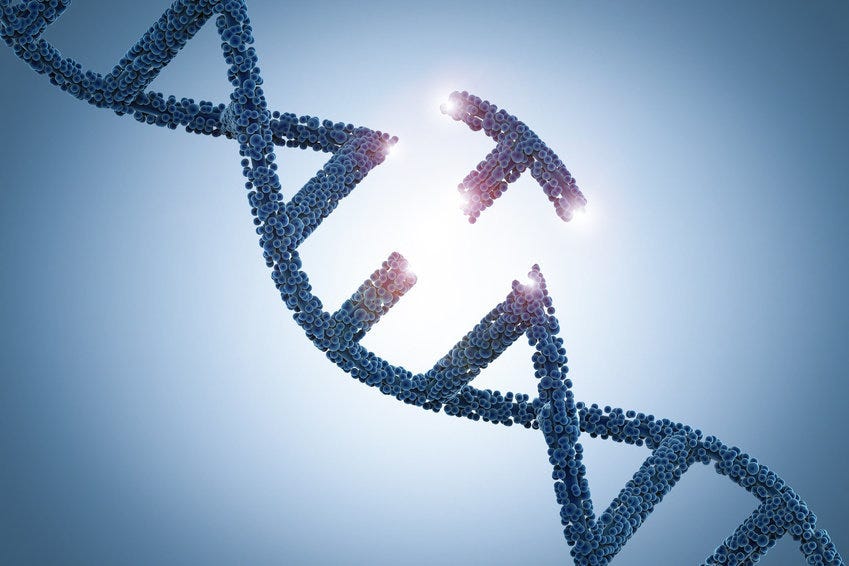
If we wish to understand CRISPR, we should go back a few decades. In 1987, a few Japanese scientists were studying E. coli when they came across unusual repeating sequences in the bacteria’s DNA. They stated that the biological significance of these sequences was unknown.
Since then, researchers found similar clusters in the DNA of other bacteria (and archaea) and gave those sequences a name: Clustered Regularly Interspaced Short Palindromic Repeats — or CRISPR (Plumer, Barclay, Belluz, & Irfan, 2018). Feng Zhang (scientist at the Broad Institute in Boston), co-authored a paper in Science in February 2013 that showed CRISPR/Cas9 to be useful in editing the genomes of cultured mouse cells or human cells. In the same issue, Harvard’s George Church and his team demonstrated how a different CRISPR technique could be used to edit human cells. Scientists can not only use CRISPR to “silence” genes by snipping them out, but they can also attach repair enzymes to substitute desired genes into the “hole” left by the snippers (though this latter technique is riskier to carry out). So, for instance, scientists could direct the Cas9 enzyme to slice out a gene that causes, say the Huntington’s disease, and insert a “good” gene to replace it (Plumer, Barclay, Belluz, & Irfan, 2018).
However, gene editing itself isn’t new. Various procedures, to remove specific genes, have been around for years. But the exceptional precision of CRISPR makes it quite revolutionary! The Cas9 enzyme goes wherever you tell it to! In the past, it may have cost thousands of dollars and even more extended periods of time to alter a gene. Now it can cost just $75 and only take a few hours. This technique seems to have worked on every organism it’s been tried on (Plumer, Barclay, Belluz, & Irfan, 2018). It is no doubt that this has become one of the fast-moving and hottest fields around.
In sickle cell disease, for example, one building block — an A — is mistakenly converted to T, in a gene that creates hemoglobin. Matthew Porteus, an associate professor of pediatrics at Stanford, and a scientific co-founder and advisory board member of CRISPR Therapeutics, compares it to “a typo in a book that contains 6 billion letters.” Porteus has spent six years trying to repair that one mutation using older gene-editing technologies. With CRISPR, he found it much easier to use and far more efficient. People with sickle-cell disease are known to have two defective hemoglobin genes in their stem cells, one from each parent. Together, these two faulty genes are the cause for red blood cells, which are normally disc-shaped and elastic, to become rigid and sticky as they mature. A proposed Stanford clinical trial will focus on the stem cells in our bone marrow that produce red blood cells (Shwartz, 2018).
In this trial, Porteus has planned to repair and replace defective blood stem cells in sickle cell patients. The idea is to transform those patients into healthy people, by converting the two abnormal hemoglobin genes, into stem cells with just a single abnormal gene. CRISPR’s job will be to get rid of the mutated DNA sequence from one of the genes. When the gene has been repaired, the newly modified stem cells with the sickle-cell trait will be introduced back into the patient’s bloodstream; some may find their way into the bone marrow and soon start cranking out millions of healthy red blood cells as well (Shwartz, 2018). According to the 2017 National Academy of Science report, sickle-cell disease seems well-suited for CRISPR gene therapy since it targets a specific type of cell.
Editas, a leader in CRISPR gene editing, reports that two versions of the approach may be useful in treating blood disorders. One is CRISPR Cas9 and the other CRISPR Cpf1; the latter said to have certain advantages than the former. The poster session at the 59th Annual Meeting of the American Society of Hematology in Atlanta in December 2017, showed that Cas9 was able to correct about 30 percent of the DNA sequence in ß-hemoglobin genes with the protein CD34 on the surface of their cells. Cpf1 nuclease was able to efficiently edit the targeted sites, underscoring its potential as a gene editing tool, researchers said. An advantage of Cpf1 is that it can edit more gene sites than Cas9 can recognize and cut (Mumal, 2018).
There is no doubt that the potential of CRISPR offers scientists promise of efficient and effective treatments for inherited diseases such as sickle cell anemia, cystic fibrosis and so on. Other applications such as editing genes of various crops, developing antibiotics, altering species and creating designer babies à la the movie Gattaca (although there seems to be an ongoing debate about the ethical value of it) are on the CRISPR path. One can only hope that this research continues to drum up funding for gaining further insights into improving our natural systems and, treat or eradicate incurable diseases at the molecular level for future generations. A positive approach for a constructive impact to society, should be the purpose for development of further testing and therapy.
A step towards changing the world, one gene at a time.